Integrating Smart Building Control Systems to Enable Grid-Interactive Buildings (GIB)
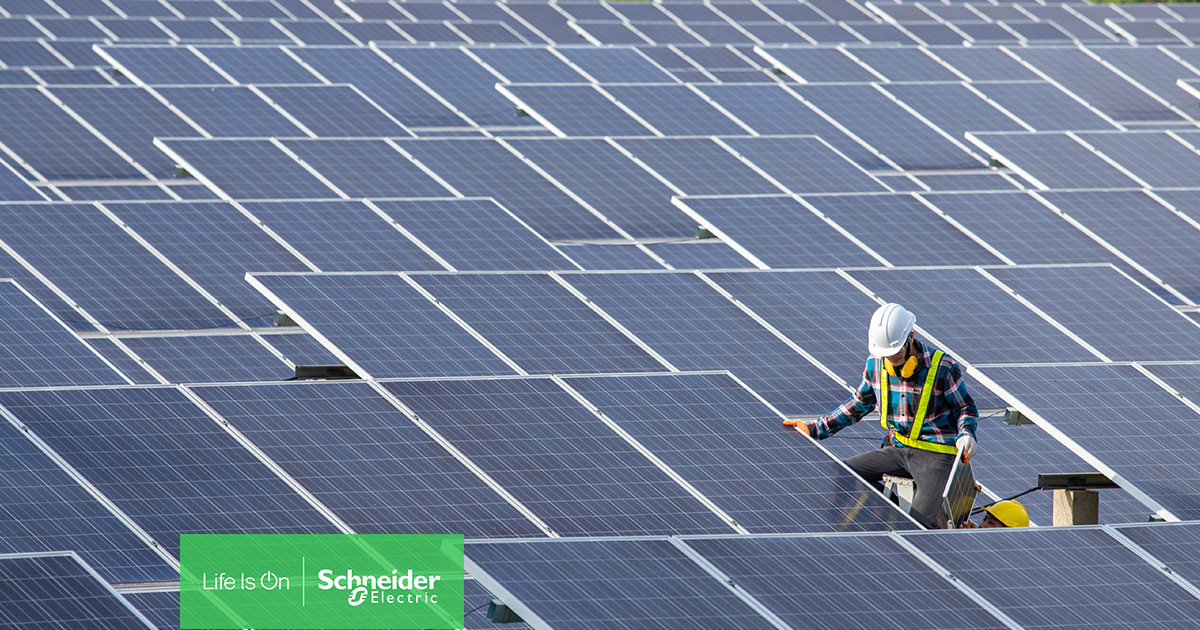
Buildings are a major contributor to global energy-related CO2 emissions. Efforts to achieve net zero emissions for buildings involve, in part, reducing overall carbon-based energy consumption and developing on-site micro-grids. Maximizing the sustainable and financial impacts of these efforts re-quires a coordination and interaction between the larger energy grids and buildings. This paper explains what it takes to make a “grid-interactive building” or GIB and presents an example architecture.
Integrating Smart Building Control Systems to Enable Grid-Interactive Buildings (GIB)
White Paper 509
Version 1
Energy Management Research Center
by Anubama Chinnakannan and Hugh Lindsay
Executive summary
-
Buildings are a major contributor to global energy-related CO2 emissions. Efforts to achieve net zero emissions for buildings involve, in part, reducing overall carbon-based energy consumption and developing on-site micro-grids. Maximizing the sustainable and financial impacts of these efforts requires a coordination and interaction between the larger energy grids and buildings. This paper explains what it takes to make a “grid-interactive building” or GIB and presents an example architecture.
Introduction
The World Green Building Council reports that building construction and operation represents nearly 40% of energy-related CO2 emissions worldwide today and still an additional 230 billion square meters of new buildings will be constructed over the next 40 years1. In interpreting this data it’s important to consider that a building exists within a larger energy system where traditionally energy has been supplied by electric and other utilities with the building acting passively as an energy consumer. In considering the pathways to a zero-carbon future, building owners and the industry itself must take a broader systemic approach and rethink the potential for a building to significantly reduce energy consumption and carbon by becoming an active participant in that energy system. The shift to full building electrification, renewable energy microgrids, energy storage, and digitally automated load control gives building operators the means to act as a ()prosumer.
A prosumer building produces and consumes energy based on the real-time conditions of the grid and the operational needs of the building and its occupants. A building that acts as a prosumer is said to be a “grid-interactive” building (GIB). The US Department of Energy estimates that “… over the next two decades, grid-interactive buildings (also known as, grid-interactive efficient buildings, or GEBs) could deliver between $100 and $200 billion in savings to the U.S. power system and cut CO2 emissions by 80 million tons per year by 2030, or 6% of total power sector CO2 emissions.”2 and similar estimates are anticipated in the rest of the world. These positive impacts will drive further adoption of building-to-grid technologies and operational procedures.
Building-to-Grid Integration for More Effi-
Building-to-Grid Integration for More Effi-
cient, Sustainable, and Resilient Energy Systems
cient, Sustainable, and Resilient Energy Systems
In Schneider Electric White Paper 517, “”, we gave a high-level introduction to the building-to-grid, or grid-interactive building, topic and considered the drivers and key enablers of this evolution. Figure 1 shows a high-level overview. In this follow-up paper, we shift the focus to address what it takes for a building to become grid interactive. This will include digitalization, as well as the integration and orchestration of the various building energy and controls systems to become a GIB.
After a brief review of the grid-interactive buildings concept and their benefits, we will highlight some of the building energy and control systems that enable improved decision making and automated responses, and we will detail the necessity of synchronized integration between these internal systems and with the external systems that similarly automate the grid. Next, we will propose a theoretical architecture and some implementation guidelines and considerations. We will conclude with a review of some existing examples of grid-interactive architectures within Schneider Elec-tric’s own real estate portfolio.
GIB concept and its benefits
Grid interactive buildings are structures capable of integrating renewable energy generational sources such as solar arrays, wind turbines, and energy storage systems into the grid. A key feature in designing these structures is data generation capabilities, hence, grid interactive buildings rely on digital metering, controls, and IoT sensor systems that generate relevant data. These network-connected technologies support data processing for decision making and automated controls that are essential for the continuous operation of building functions in sync with the real-time conditions of the grid. GIBs support grid-scale, efficient operation by employing on-site distributed energy resources (DER) and curtailable loads. The key to enabling grid interactivity lies in the interoperability between the building management system
(BMS), microgrid management system (like management systems for solar arrays and energy storage), and power management systems (or electrical power management systems, EPMS). Full, real-time grid interactivity also requires a standardized exchange of data with grid automation systems like Advanced Distribution Management Systems (ADMS) and Distributed Energy Resource Management Systems (DERMS).
2021 BTBC Executive Summary 2021.pdf
A National Roadmap for Grid-Interactive Efficient Buildings (lbl.gov
Figure 1
Grid-interactive building concept

InternalGIBs support grid services
The grid is under a lot of demand from increased building electrification, EV charging, urbanization, and innovation. This pressure can be reduced by utilizing buildings that are capable of flexible load operation. This can be achieved by shedding nonessential loads during peak consumption or shifting major loads to different times. This will help reduce demand on the grid and lower building operating costs. Common industry practices that are employed to reduce the demand on the grid are:
- Load Shedding: With energy data from power meters and load controls via the BMS, a grid interactive building can shed its load from the grid. The building can opt to compensate by using on-site generation assisted by the microgrid controllers. This helps smooth power peaks and reduce demand on the grid when it is overloaded. This is done by shedding non-essential loads during times of peak consumption.
- Load Shifting & “time of use” (TOU) contrasts with the above bullet in that there is a planned schedule that allows the building to use or power major loads at a different time. For example, if there is a predicted demand charge at 9am, a building can choose to turn on its heating or cooling systems an hour earlier and allow coasting. This type of use allows a reduction in building operating costs by avoiding demand charges or TOU peaks and can help avoid periods of higher carbon intensity (where real-time carbon intensity data is available).
Energy arbitrage is possible when we have a time-of-use (TOU) tariff with variable prices during the day. This is aided by on-site generation systems.
Load flexibility (or demand flexibility) initiatives support the grid by helping to achieve a reduction in generation costs over the long term. This would be achieved through
Figure 2
RMI
RMI
Grid-interactive building load profiles illustrated by
delaying capacity investments, optimizing grid resources, and reducing operation and maintenance costs for utilities as power generation becomes increasingly decentralized.
GIBs benefit building owners and occupants
In addition to the benefits of GIBs described above, there are applications and associated benefits that accrue directly to the building stakeholders such as improving sustainability.
This diagram (Figure 2) from the Rocky Mountain Institute (RMI) highlights the impacts that Load Flexibility offers to help buildings become more energy efficient and resilient. By using smart building controls, such as occupancy-based lighting, solar tempering, thermal enveloping, and optimized energy modeling, buildings can reduce their energy use. Adding solar PV to the roof can make the building net-zero energy or even net-positive. Integrating and coordinating power, building, and mi-crogrid systems can optimize energy and load across all systems, while still prioritizing occupant comfort.
Note: This system can be extremely occupant centric, in the sense that it can prioritize occupant comfort over energy efficiency or resilience, but it can also prioritize resiliency and efficiency in the condition where the grid is under extreme stress. The key is having the right information and the right granularity of control to make informed decisions in real time.
Optimized GIB management
While managing demand response for a building, a GIB’s management systems will link and coordinate together various data streams including:
- building occupancy levels
- building usage patterns
- status of loads required for critical system operation
- DER production based on sunlight or wind conditions
- weather conditions for load estimations, building energy, and grid demand
These data streams can be used as historical datapoints to create a strong prediction engine. Additional applications can be added onto this structure to add additional services such as tariff arbitrage. For example, building owners can receive incentives for participating in net metering or demand response programs that are in addition to saving costs by reducing energy consumption and avoiding high peak charges. This is usually supported by Microgrid Control Systems.
Integrated systems produce data that can be used for AI and Machine Learning algorithms. This creates the potential for improved building automation and control.
The data points required for implementing load flexibility use cases are:
- building energy and load metrics
- open-source data for weather
- solar irradiance
- energy tariffs
- occupancy planning
Smart control system integration
Figure 3
EcoStruxure Power Monitoring Expert for building energy mapping, analysis, and estimations
For a building to become grid flexible, an integration and coordination between 3 software management systems (or “smart control systems”) within the building is required for full optimization:
- Electrical Power Management System (EPMS)
- Building Management System (BMS)
- Microgrid Control Systems (MCS), where on-premise renewable energy generation is present
Electrical Power Management Systems (EPMS)
EPMSs collect, store, and deliver all energy meter data for critical decision making done by the BMS and MCS. The EPMS includes meters and software. These systems are used for power quality, power factor, harmonics monitoring, and supports voltage and frequency regulation. Figure 3 shows an example screenshot of energy consumption data by location for a Schneider Electric building.

Today, power management software, as shown in Figure 3, is enabled by power meters that are network connected, which are sometimes called, “smart meters”. When these meters feed data to EPMS software tools, operational decisions improve to reduce consumption, cost, and emissions. Some smart power meter models provide the required data outputs needed to participate in TOU and load-shift
applications. Smart meters can be connected to solar or other DER feeds to measure and analyze various degrees of data and its impact on carbon emissions.
Power management and data logging are valuable in the scope of developing AI-enabled energy optimization engines. Power data integrated natively into the building's systems unlock key use cases, such as:
- Asset management and AI enabled energy optimization with a live response variable (e.g.: power consumption)
- Fault/anomaly detection and root cause identification at sub-circuit-level based on power & building data
Building management systems (BMSs)
BMSs are comprehensive software and hardware-enabled control layers that provide monitoring and control functions for mechanical and electrical equipment, as well as providing indoor environmental monitoring and determining the building’s overall energy footprint. A BMS manages individual systems such as HVAC, lighting, access control, fire, and security among others. The block diagram below (Figure 4) is a high-level representation of the hardware, software, and communication protocols that make up a BMS for a Schneider Electric site.
This site’s BMS suite visually represents flexible and controllable HVAC and lighting installations, zone monitoring and control, and a dense IoT network of sensors that acts as a data resource. These data points represent things like building occupancy levels, indoor environment quality, lux, decibel levels, people count, people flow, etc.
Figure 5 shows the granularity of zone control on one section of one floor in the building. This is beneficial in applications that support zero net-energy efforts such as demand controlled ventilation (DCV)3, electrified building operation, and flexible load control. This site also demonstrates (in Figure 6) the BMS’s ability to tap into its EPMS counterpart and pull data as needed –this avoids replicating and rehousing all these data points.
Attributes of an Effective Maintenance Program for Data Center Physical Infrastruc-
ture
Figure 4
Building management system – block diagram with representation of zone control

Figure 5
Building management system – screenshot of floor plan depicting individual zone climate monitoring and controls

Figure 6
BMS platform showing the chilled water system. The chiller trend data is imported from the power management system using EcoStruxure Web-Services. A typical approach uses Modbus TCP comms protocols. This image demonstrates the BMS’s ability to interface with the EPMS data captures.
Microgrid control systems (MCS)
Sometimes known as a microgrid energy management system (MEMS), MCS is also a software-based management system, with connections to ground controllers that act as enablers for data and control communication inputs and outputs. It manages all on-site DER assets. The building or utility operator does not look behind the scenes at individual PV panels, wind turbines, battery storage units, or EV charging stations. The Microgrid operating platforms take care of system-level management and provide information via virtual power plants to and from systems such as the grid or BMS. The microgrid system by itself can facilitate grid support such as net metering and demand response. It can work with the BMS as a load to carry out load shedding, flexibility, TOU, and frequency regulation. Commercial sites can benefit from DER and BMS integrations especially with use cases such as optimal start and stop of HVAC systems, tariff arbitrage, and demand-controlled energy use.
The block diagram shown in Figure 7 is a high-level representation of a microgrid control system and their communication protocols. Instead of just acting as an added power resource, it is interesting to see connections to HVAC systems (by tapping into the BMS) and the utility for the applications mentioned above.
Figure 7
Block representation of a DER management system
Figure 8
Microgrid overview dashboard for a Schneider Electric site

Schneider Electric’s IntenCity office building in France
Schneider Electric’s IntenCity office building in France
is an example of a site that is using active net-metering with the local grid. The site’s DERs, Solar PV and Wind generate enough power, on most days, to cover the site and with the excess energy it decides to either charge the battery storage system or deliver power to the local grid.
Figure 8 shows a live screenshot of the DER facilities and power distribution systems at a Schneider Electric office:
In a building with on-site DERs, grid interactivity can be thought of as ‘the integration and continuous optimization of DERs for the benefit of building owners and occupants, as well as the grid’.4
Microgrids and distributed energy resources can also be leveraged similarly with Virtual Power Plants to aggregate DERs to help balance the larger grid through the provision of services such as demand response or frequency regulation. If combined, VPPs and microgrids can optimize buildings and the surrounding grid network to meet both sustainability and economic goals. For example, VPPs could be
Conceptual mapping and understanding GIB layouts
Figure 9
Block diagram of interoperability between necessary systems showing a theoretical depiction and electrical interface between these systems
VPP software
VPP software
created by aggregating multiple microgrids, tapping these fleets for DERs for data to optimize operations for resiliency and environmental benefits. Microgrids can also use to access the grid when they need to supplement their own on-site power generation.
These typically, independently operated and maintained systems (i.e., the EPMS, BMS, and MCS) need to have a common data and control layer to begin operating in conjunction with each other. This integration opens the potential for multiple use cases, applications, and solutions that were discussed previously. Thus, for a building to be fully grid-interactive, we need to integrate and coordinate three smart building control systems: the EPMS, BMS, and the MCS.
This section describes a generic layout that achieves system to system interoperability. While many sites have achieved digitalization, automation, and data-driven operation, the next step to realize the full potential of these efforts lies in interoperabil-ity/coordination of these management systems.

A unified system will benefit from connecting with all the right data and communication modules in a single repository. This repository can be a local or cloud database and is represented by the “System Interface” in Figure 9. Data sharing between the siloed systems is essential to carry out GIB applications and critical in several areas of operation such as power synchronization between them. Figure 9 is a depiction of this unified system operation. The automation system can represent a home/building/industry BMS that is connected to a microgrid or any form of DER. The same goes for the utility management system and associated grid-scale DER connection. While each system is necessary to oversee monitoring, controls, maintenance, and core functionality within itself, a GIB will benefit from the presence of a systems interface to oversee real-time, single-entry data points from each system (e.g., energy generated, stored, required, and received).
Figure 10
Cross-sectional theoretical layout of different GIB systems
Within a GIB, building automation expands beyond traditional capabilities to include automation of on-site generation & storage assets, data, as well as control communications to and from the grid (or connected community, if part of one), and gather and digest critical data from third-party sources. These data sources could be energy prices that is used to carry out time of use (TOU) optimization and tariff arbitrage, weather for DER optimization and load flexibility, grid demand and supply capabilities for automated demand response (ADR) support.
These functions do not require building modules within the BMS to tackle power management and DER management. This requires connectivity that allows the BMS to push and pull data and control points between the EPMS and DER systems. Today, applications such as demand flexibility and alternate TOU are achievable via independent building system controls. Although some level of grid-interactivity is possible with just the BMS, a GIBs value significantly increases with system integration that provides timely responsiveness and general flexibility.
A key aspect of this integration of control systems is the ecosystem of IoT devices, or “connected products”. These devices may already exist in commercial buildings, and if not, are a relatively cost-effective investment and can be leveraged into the GIB vision. Typical connected IoT devices within a building in this sense are:
- occupancy sensors whose data points can be used for electrical load management
- room sensors monitoring indoor environment quality such as temperature, humidity, sound, and lighting used for demand-controlled HVAC and lighting system operation
- actuators and controllers to support flexible HVAC optimization
- smart meters for power distribution monitoring and control
In addition, innovation in sensor technology has made investments in setting up this infrastructure easier and more cost effective with non-invasive multi-sensors. GIB architectures need to be defined by creating and interconnecting ‘Data Technology Layers’ and ‘Physical Systems’.

The proposed GIB layout shown in Figure 10 consists of three layers: 1) Connected Products, 2) Edge Control and 3) Apps & Analytics layer.
The Connected Products layer resides in the grid-interactive building (GIB) and consists of two sets of devices: 1. Data sources – these devices deliver data points for decision making that translate to control algorithms (some examples are defined above); 2. Controllable equipment – these are HVAC and lighting systems that are operated by the controllers (fed with control points as stated above). These connected products give way to an edge operation and control layer that can act as a medium for gathering, extracting, and controlling the data points. Information is communicated to the BAS via open or custom application programming interfaces.
The Edge Control Layer is primarily composed of a BAS/BMS that is centrally supervised by building facility managers. The data layer enables automation in the form of regular and remote monitoring and controls. It also provides the ability to get these systems connected to the cloud, unlocking the true potential of IoT, big data and AI. It can enable this automation with scripts, schedules, and AI applications. The GIB Apps & Analytics layer will perform advanced data analytics using data-driven machine learning (ML) algorithms to provide actionable intelligence to building facility managers in the form of optimal HVAC set points, minimum air flow rates, control schemes and load forecasts as well as energy efficiency and load-shedding and shifting opportunities, based on internal and external data point analyses. The recommendations will ensure occupant preference and comfort is always maintained while meeting the global optimums.
The Apps & Analytics Layer can also host aggregator agents (software) for various applications. For example: an interaction with the grid operator for dispatching real (P) and reactive (Q) power from the GIBs and synergistic DERs as well as provide the ancillary grid services. All information exchange between the Supervisory and Control Layers as well as from Cloud resources will be via application programming interfaces (APIs; in this case, RESTful APIs).
Such information exchange functionality is now present in many connected products such a smart thermostat. Some water heaters use the CTA-2045 communication interface standard for these applications. These are examples of direct building module to grid connections for the benefit of flexible load operation.
Maximizing the full effect of the entire Data Technology layer might require upgrades to existing physical systems. For example, state of the art sensing and control capabilities for HVAC and lighting systems provide opportunities for hourly load management, improved control flexibility based on zone occupancy, CO2 levels, temperature distribution, occupant feedback, etc. Strategies such as pre-cooling/pre-heating a building space, coasting through a period or shift in HVAC TOU can take data points for occupant comfort into the decisional making structure too.
A GIB will benefit from being able to provide more sensor data points than a traditional building. Any data point that might have a direct load flexibility impact should be considered essential while architecting the system. Examples are occupancy, lux levels, thermostats, humidity, air quality and pressure monitoring for every controllable zone.
The connection to the grid, or more specifically a network or independent system operator can be at the Edge or Analytics layer. We need to keep in mind that this connection involved an active two-way power flow and communication stream for data and controls.
Schneider Electric’s sites use Machine Learning algorithms for forecasting site generation and consumption along with estimated emissions reductions. With available data streams from the BMS, EPMS, and MCS can create an intelligent analytics
Figure 11
This screenshot shows a forecasted and actual site load and energy consumption; solar irradiance forecasts and actual production; offsets in terms of CO2 emissions
engine that supports energy, cost, and carbon impact minimization. The Schneider Electric building draws load data from the EPMS and understands building needs to send decisions on DER management back to the BMS. This analytics layer comprises of active forecasting models for: Building loads, Energy Tariffs and Indoor and Outdoor Temperatures.
We need to maximize the benefits of an Apps & Analytics layer that houses the previously independent operating systems to achieve further optimization of building and GIB systemic operation.
The benefits of tightly integrating power management and DER management into the building management (at the edge and in the cloud) as above will help you:
- accomplish sustainability goals (energy management)
- maximize resiliency (including fault-detection and resolution in both mechanical and power domains)
- be hyper-efficient with operations and maintenance via more intelligent automation (Edge control systems) and analytics for enhanced decision support and predictive maintenance (Apps & Analytics layers)
- do all of this without compromising on occupancy safety and comfort
The integration above should also carry a User Interface with the GIB which can be in various forms and for various applications such as Human interference, Human-Machine interactions, and even just informative displays.
Conclusion
Grid interactive buildings (GIBs) are designed to integrate renewable energy generation sources into the grid, and rely on data generation capabilities, digital metering, controls, and IoT sensor systems to process data for decision making and automated controls. GIBs support grid-scale efficient operation by employing on-site distributed energy resources (DER) and curtailable loads, and require interoperability between the building management system, microgrid management system, and power management systems for real-time grid interactivity.
GIBs can reduce the pressure on the grid from increased building electrification, EV charging, urbanization, and innovation by shedding non-essential loads during peak consumption or shifting major loads to different times. This helps reduce demand on the grid, lower building operating costs, and supports load flexibility initiatives that
help achieve a reduction in generation costs over the long term. GIBs also offer direct benefits to building stakeholders such as improved sustainability, occupant comfort, and energy efficiency by using smart building controls, solar PV, and coordinated power, building, and microgrid systems.
Maximizing the energy efficiency and sustainability benefits of grid-interactive buildings requires an integration and coordination of the building’s BMS, EPMS, and MCS. This paper explains this integration and coordination and shows some high-level architectures for how this can be accomplished today.
About the authors
-
-
-
Anubama Chinnakannan is an Application Engineer in the CTO Office of Digital Buildings at Schneider Electric. She received her bachelor’s degree in Electrical and Electronics Engineering from CEG - Anna University, India and her master’s degree in Energy Systems Engineering from Northeastern University, USA. She is a WELL Accredited Professional and Certified CESAM Associate. She actively works on data experimentation, thought leadership material for Artificial Intelligence in buildings, grid-interactive efficient buildings research, multi-technologies research and innovation, and evaluations of startups in the buildings domain.
-
-
Hugh Lindsay is the Director, Digital Ecosystems and Architectures for Enterprise Solutions at Schneider Electric. He holds a Bachelor of Applied Science in Communications from Simon Fraser University in Canada and a master’s certificate in Systems Architecture from CESEAMES Polytechnique in France. Over his 20+ year career, Mr. Lindsay has held numerous management and senior leadership roles related to the energy, sustainability, resilience, and operational efficiency of digital systems for Smart Buildings in Commercial Real Estate, Government, Finance, Colocation and Internet Giants, Advanced Education, and Industrial sectors.
RATE THIS PAPER
RATE THIS PAPER
Building-to-Grid Integration for More Efficient, Sustainable, and Resilient Energy
Building-to-Grid Integration for More Efficient, Sustainable, and Resilient Energy
Systems
Systems
White Paper 517
Browse all
Browse all
white papers
white papers
whitepapers.apc.com
whitepapers.apc.com
Browse all
Browse all
TradeOff Tools™
TradeOff Tools™
tools.apc.com
Note: Internet links can become obsolete over time. The referenced links were available at the time this paper was written but may no longer be available now.
Contact us
For feedback and comments about the content of this white paper:
Schneider Electric Energy Management Research Center dcsc@schneider-electric.com
© 2023 Schneider Electric. All rights reserved.